E-Archive
Science Update
in Vol. 20 - September Issue - Year 2019
Comparison of Shot peening, Cavitation Peening and Submerged Laser Peening on Improvement of Fatigue Strength of Additive Manufactured Metal
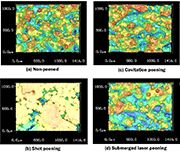
Fig. 1: Aspect of specimens manufactured by electron beam powder bed melting (EBPB)
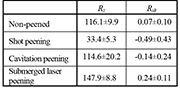
Table 1. Surface characteristics of specimens
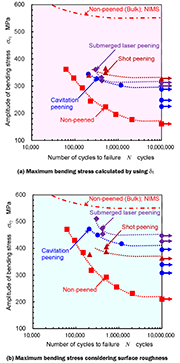
Fig. 2: Improvement of fatigue strength of Ti6Al4V manufactured by EBM by means of mechanical surface treatment
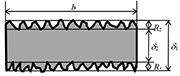
Fig. 3: Schematic diagram of thickness of specimen for calculation of bending stress
Introduction
Additive manufactured AM metals are attractive materials for medical implants and aviation components, as shapes are formed directly from CAD data and the other advantages. However, fatigue strength of AM metals is considerably weak comparing with that of bulk metals. Thus, enhancement technique of the improvement of fatigue strength of AM metals is required.
The popular method for the improvement of metallic materials is shot peening, and the enhancement of AM metal by shot peening has been demonstrated [1]. On the other hand, novel peening methods such as cavitation peening using a cavitating jet and submerged pulse laser have been proposed [2]. In the case of cavitation peening, cavitation bubble impacts is used to introduce plastic deformation pits without shots; thus, chemical cleaning after shot peening is not required. And also, the impact of cavitation bubble can peen narrow regions in the bottom of un-melted particles on the surface of AM metals, as the shock-wave induced by bubble collapse is used.
Recently, the comparison between the improvement on the fatigue strength of stainless steel SUS316L by cavitation peening, water jet peening, shot peening and submerged laser peening was investigated, and it was reported that the highest fatigue strength was obtained with cavitation peening, followed by shot peening, laser peening and finally water jet peening [3]. Note that a novel mechanical surface modification method to improve the fatigue strength of AM metal using a cavitating jet with abrasive was proposed by the collaboration work with Tohoku University and Boeing [4].
In the present paper, the enhancement of fatigue strength of AM metal by cavitation peening compared with shot peening and submerged laser peening was demonstrated [5].
Material and Methods
The used material was titanium alloy Ti6Al4V, and the specimens were manufactured by electron beam melting (EBM). The averaged diameter of the used particle size at EBM was about 75 μm. The spot size of the electron beam was 0.2 mm in diameter and the stacking pitch was 90 μm. The specimens were heat-treated at 1208 K under vacuum for 105 minutes then cooled in argon gas. After that, aging was carried out at 978 K under vacuum for 2 hours, followed by cooling in argon gas.
The fatigue specimens were treated by cavitation peening using a cavitating jet, submerged laser peening and shot peening. In the case of each peening method, the condition at each peening were chosen by considering the previous paper [3]. In the case of cavitation peening, the cavitating jet was used. The injection pressure of the jet was 30 MPa and the nozzle throat diameter was 2 mm, with distance from the upstream corner to the specimen surface being 222 mm and processing time per unit length being 10 s/mm.
At the submerged laser peening, Nd:YAG laser with Q-switch was used. The maximum energy and the pulse width of the laser were 0.35 J and 6 ns. The used wave length was the fundamental harmonic, i.e., 1,064 nm, as impact at bubble collapse that was developed after the laser ablation was used [3]. Note that the impact induced by bubble collapse was larger than that of laser ablation [3], with pulse density per unit area being 5 pulses/mm2.
In the case of shot peening, the specimen was treated by recirculating shot accelerated by a water jet [6]. The injection pressure of the jet was 12 MPa, and the processing time per unit length was 1 s/mm.
The specimens with and without peening were tested by a conventional Schenk-type displacement controlled plane bending fatigue tester at R = -1.
Aspect of Specimens Manufactured by Electron Beam Melting
Figure 1 shows the aspect of specimens of non-peened, shot peening, cavitation peening and submerged laser peening observed by a laser microscope with 3D stacking. In Fig. 1, the blue color reveals valleys and the red color shows the top of particles. Table 1 reveals maximum height of roughness profile Rz and skewness Rsk in order to show the surface characteristics of the specimens. As shown in Fig. 1 (a), a lot of un-melted particles were observed on the surface. When the surface was treated by shot peening, the particles were deformed as shown in Fig. 1 (b) and Rsk was minus value. However, deep valleys were observed, and these could not be removed by the shot peening. On the other hand, for the treated surface by cavitation peening and submerged laser peening, the un-melted particles were still observed; namely, the peened surfaces were scarcely changed. In the case of cavitation peening, Rz was slightly decreased; however, Rz of submerged laser peening was larger than that the of non-peened one, as the laser pulses produced plastic deformation pits systematically, then the sequential pulses formed a kind of crest.
Improvement of Fatigue Strength of Specimens Manufactured by EBM
by Means of Peening Methods
Figure 2 shows the result of a plane-bending fatigue test for non-peened, shot peening, cavitation peening and submerged laser peening [5]. As the specimen surface was considerably rough as shown in Fig. 1 and Table 1, Rz was considered to calculate the maximum bending stress. In Fig. 2 (a), the maximum bending stress was calculated by using the thickness δ1 as shown in Fig. 3. However, the layer shown by Rz in Fig. 3 was a kind of unsupported layer. Thus, δ2=δ1- 2×Rz was used in Fig. 2 (b).
In Fig. 2 (a), the fatigue strength of shot peening was largest, as the top of un-melted particle was deformed. When the fatigue strength at 107 was calculated by using Little’s method [7], it was 169 ± 8 MPa for the non-peened samples. Note that the fatigue strength at 107 of bulk material made of Ti6Al4V with heat treatment was 545 ± 10 MPa [8]; namely, the fatigue strength of titanium alloy Ti6Al4V manufactured by EBM was considerably weak compared with bulk material. When the titanium alloy Ti6Al4V manufactured by EBM was treated by peening, the fatigue strength was 335 ± 6 MPa for shot peening, 296 ± 8 MPa for cavitation peening and 317 ± 4 MPa for submerged laser peening. All of these were drastically improved by peening comparing with non-peening; however, fatigue strength was still weak compared with the bulk material.
As mentioned above, the maximum bending stress was re-calculated considering the surface roughness. The S-N curves are shown in Fig. 2 (b). The fatigue strength using the data in Fig. 2 (b) was 221 ± 11 MPa for non-peened, 371 ± 7 MPa for shot peening, 406 ± 11 MPa for cavitation peening, and 450 ± 3 MPa for submerged laser peening. Namely, after submerged laser peening, the fatigue strength was improved about two-fold compared with non-peening, and it was about 80 % of the bulk material.
Conclusions
In order to demonstrate the enhancement of fatigue strength of additive manufactured AM metallic materials by mechanical surface treatments, the titanium alloy Ti6Al4V manufactured by electron beam melting EBM was treated by shot peening, cavitation peening and submerged laser peening, then tested by a displacement controlled fatigue test. It was revealed that the fatigue strength at 107 considering the surface roughness was improved two-fold by submerged laser peening compared with that of the non-peened samples.
Acknowledgement
This work was partly supported by JSPS KAKENHI Grant Number 17H03138 and 18KK0103.
References
[1] P. Edwards, A. O'Conner, and M. Ramulu, "Electron Beam Additive Manufacturing of Titanium Components: Properties and Performance," Journal of Manufacturing Science and Engineering-Trans.ASME, vol. 135, no. 6, pp. 1-7, 2013.
[2] H. Soyama, "Key Factors and Applications of Cavitation Peening," International Journal of Peening Science and Technology, vol. 1, no. 1, pp. 3-60, 2017.
[3] H. Soyama, "Comparison between the Improvements Made to the Fatigue Strength of Stainless Steel by Cavitation Peening, Water Jet Peening, Shot Peening and Laser Peening," Journal of Materials Processing Technology, vol. 269, pp. 65-78, 2019.
[4] H. Soyama, and D. Sanders, "Use of an Abrasive Water Cavitating Jet and Peening Process to Improve the Fatigue Strength of Titanium Alloy 6Al-4V Manufactured by the Electron Beam Powder Bed Melting (EBPB) Additive Manufacturing Method" JOM, vol. 71, no. 12, pp. in press, 2019 DOI: 10.1007/s11837-019-03673-8.
[5] H. Soyama, and Y. Okura, "The Use of Various Peening Methods to Improve the Fatigue Strength of Titanium Alloy Ti6Al4V Manufactured by Electron Beam Melting," AIMS Materials Science, vol. 5, no. 5, pp. 1000-1015, 2018.
[6] A. Naito, O. Takakuwa, and H. Soyama, "Development of Peening Technique Using Recirculating Shot Accelerated by Water Jet," Materials Science and Technology, vol. 28, no. 2, pp. 234-239, 2012.
[7] R. E. Little, "Estimating the Median Fatigue Limit for Very Small Up-and-Down Quantal Response Tests and for S-N Data with Runouts," ASTM STP, vol. 511, pp. 29-42, 1972.
[8] J. National Institute for Materials Science, "Data Sheet on Giga-Cycle Fatigue Properties of Ti-6Al-4V (900 MPa Class) Titanium Alloy," NIMS Fatigue Data Sheet No. 92, pp. 1-12, 2004.
Department of Finemechanics
Tohoku University
6-6-01 Aoba, Aramaki, Aoba-ku, Sendai
980-8579, Japan
E-mail: soyama@mm.mech.tohoku.ac.jp
